SUNDAY, 5 MARCH 2017
One of the most exciting fields to emerge in the life sciences and biotechnology in recent years is tissue engineering, which centers around creating a reliable supply of functional tissue that will not be rejected by potential transplantees. Yet if it is ever going to realise its goals in the clinic, tissue engineering will have to apply the very best that cellular therapy techniques can deliver, and combine this with a physical structure which is able to leapfrog the ordinary course of developmental anatomy: supporting, moulding, and inducing cells to form a functioning tissue.Here, we have a symbiotic relationship between two scientific disciplines. New tissue for patients would be a pipedream without the recent, and continued improvement of immature cell lines that can grow into the sort of cells they would need. Equally, we can only hope to see engineered tissues grafted into patients if there is progress in tissue scaffold technology. This is because we cannot just add a soup of cells to fill up a wound; physical structure is essential. Such will be the result of progress in the production, formulation, and shaping of biomaterials and artificial polymers, chains of repeated molecules; all with an eye toward improving the interactions of these designed ‘extracellular matrices’ (ECMs), the network of molecular ‘cement’ that provides support for all cells in the body, with delicate stem cells.
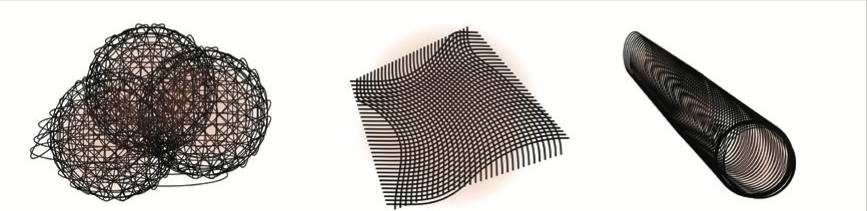
The transplantation in 2010 of a decellularised donor trachea into a 9-year old patient at Great Ormond Street Hospital, London has been validated as a successful procedure in numerous followup studies. It demonstrated that appropriate stem cells could indeed penetrate donor ECM, and moreover, stem cells can be induced to take on an appropriate tissue role, in response both to the structure in which they found themselves, and even to the air that passes by that particular organ.

The ECM in living tissues is built and maintained by the cells it holds, so it comes in many shapes and chemical compositions. What all static tissues have in common is a web of collagen and hyaluronic acid fibers, several hundreds of nanometers in diameter. These plain, inactive fibers may not be worth producing, because they would be an inferior alternative to decellularised donor tissue. To improve on donor tissue, it will be necessary to have the scaffold release embryonic steroids or identity-assigning peptides called morphogens onto the cells.

The most promising way to form polymer nanofibers at that scale is through various ‘electrohydrodynamic’ techniques, which employ electrostatic forces to stretch, dry, and even align artificial or natural polymers. Among these, electrospinning is especially versatile: it can encapsulate molecules, and turn fluidic solutions into structural fibers. By extruding one fluid inside the other, it can create aqueous, morphogen-filled cores wrapped in biodegradable polymer shells whose thickness determines the rate of release.
The electrospinning technique may well have the scope to developed into a viable tissue scaffold method. Such a scaffold would most likely comprise structural fibers and non-structural morphogen-eluting products, such as electrosprayed nanoparticles, which are produced if the unformed fiber repeatedly splits as a result of low viscosity. There are many challenges to overcome. The key weakness of these electrospun fibers in tissue engineering is their tendency to form mats where the fibers are too close together for cells to penetrate. Sacrificial fibers, made of very biodegradable materials such as polyethylene oxide, have been shown to create a void space for proliferating cells to eat through and inhabit. Whether tissue or precursor cells can avoid significant stress as a result of the breakdown of large quantities of `sacrificial fiber` polymer remains to be seen.
One solution to the cell permeation problem might be to produce cell-imbued fibers. Research at University College London and Imperial College has demonstrated how electrospinning might achieve this. Instead of signalling cells to proliferate, it appears that whole cells may be electrospun into fibers, where they can either be laid directly over tubular elements, or interspersed with other structural fibers.
Research at Tokyo university has investigated cell growth over ‘nanofibrous microcapsules,’ which are modular, stackable spheres of polylactic acid nanofibers formed through a special variation of the electrospray process, in which a very large core of vaporising water ‘blasts holes’ through the thin polymer shell. This method provides plenty of space for cellular growth, and cells avoid harmful contact with the ends of fibers. However, the deposition of drug encapsulations may be difficult, and it is far from certain whether normally functioning tissues could be grown over fibers arranged in this way.
With these opportunities and challenges in mind, it is likely that progress in artificial scaffolds will be fruitful, yet slow. Over the past two years, there has been a shift in attention from big organ scaffolds, to minimal scaffolds with interesting invasive and immunosuppressant properties, to prevent attack from the patient’s white blood cells. A very recent study, announced at the beginning of this year by a team in MIT, showed how drugged polymer encapsulation technologies, which enabled the introduction of xenogenic pancreatic islets cells in mice with minimal immunosuppressants were extraordinarily effective in tackling a mouse model of diabetes.
Nanofibrous tissue scaffolds have played a crucial, albeit more oblique role in other exciting developments, not least of which might be the work of a team at the Key Laboratory for the Biological Effects of Nanomaterials in Beijing, who used polymer nanofibers as the template for grooves which guide neuronal growth in straight lines: possibly over electrical appliances; possibly as an excellent robotic interface in prosthetics.
All of these biotechnologies will inevitably rely on the concurrent development of cell, gene therapy and transfection methods, so that they can become both medically acceptable, more commercially viable, and safer. As these advance, a lack of decellularised donor organs may ultimately prove to be a major impediment to medicine and research. For this reason if nothing else, tissue scaffold technology deserves continued attention.